The heart of future quantum devices could be illuminated by arrays of tiny glow sticks, developed by physicists at the Australian National University.
A group from the Department of Electronic Materials Engineering (EME) has fabricated an array of tens of thousands of indium phosphide nanowires and made them glow by incorporating quantum point emitters into them.
While similar devices have been created before, the team has developed a revolutionary fabrication method that is accurate and fast enough to allow scale-up of chip fabrication.
“Our strategy produces single quantum dots in nanowires with excellent crystalline quality, optical performance, and highly efficient performance,” said Ms. Xiaoying Huang, first author of the paper published in the journal ACS Nano, and a member of the EME and Biochemical Research Institute. ARC Center of Excellence in Transformational Optical Systems (TMOS).
“Our method is also much faster – we developed the array directly in a few hours, whereas other research groups using different methods could take weeks to place single quantum dots into cavities.”
Single quantum dots are very small (about 13 nanometers in diameter), which creates better quantum confinement of single photon sources, which is essential for devices based on quantum information transfer.
Quantum dots have been used before to make single photon sources, but the challenge has been to make these small light sources bright enough, in a way that enables inevitable large-scale manufacturing. Coating them with nanowires addresses this challenge: the internal reflection of the nanowire forms an optical cavity around the quantum dot, increasing the intensity of the emitted light.
Initially, physicists tried to fabricate optical cavities by etching, that is, carving shapes out of a larger piece of indium phosphide that had quantum dots embedded in it. However, the synthesis process randomly distributed the quantum dots, which made locating them and then drilling cavities around them difficult and time-consuming. The quality was also unreliable, as engraving left irregularities on the side walls of the bores that compromised emission.
Instead, the EME team decided to turn their approach on its head and grow nanowires from the ground up, using a process called doping, in which atoms are deposited from vapor. While the team had already mastered the technique of precisely growing arrays of hexagonal indium phosphide nanowires, they faced the challenge of how to integrate single quantum dots within the nanowires.
Ms. Huang explored droplet nucleation, a technique in which indium droplets are formed on prefabricated nanowires.
First, I found that multiple droplets would deposit at the end and along the nanowire. However, by shortening the nanowires, and reducing the droplet deposition time to just 0.25 seconds, they were able to reliably deposit a single droplet onto each nanowire in a 90 μm x 90 μm matrix.
These droplets are only about 48 nanometers in diameter and 13 nanometers in height. They are then crystallized into quantum dots (about 13 nanometers in diameter and about 4 nanometers in height) by adding phosphorus and arsenic to the vapor, converting the drop into indium arsenide phosphide, a quantum dot that emits light in the near infrared (about 900 nanometer wavelength). ).
Finally, another round of indium phosphide deposition is performed, which encapsulates the quantum dot, increasing the lengths of the nanowires from 3 to 4 microns, and their diameters from 50 to 400 nanometers.
The final product can be excited with a red laser to make the quantum dots emit photons one by one, with this emission enhanced by the coated nanowire that acts as an optical cavity around the quantum dot.
At best, with just excitation of the nanolaser, the resulting emission was in the millions of numbers per second, a low gravitational rate.(2)(0) with a value of 0.05, a short lifetime of 350 picoseconds, and a Purcell enhancement factor of three.
However, not all nanowires perform optimally – while nanowire cavities can be fabricated reliably and produce consistent resonances to the cavity wavelength, the quantum dot emission wavelength varies. This is a result of variation in the size and composition of quantum dots and can cause a mismatch between the quantum dot emission wavelength and the strongest cavity mode of the nanowire, limiting the emission intensity.
Ms. Huang believes she can improve the quantum dot manufacturing process to increase reliability. She also performs simulations to explore different cavity geometries alongside nanowires.
“Although it is challenging from a manufacturing point of view, incorporating quantum dots into geometries such as rings or disks can give us a much higher Purcell factor,” she said.
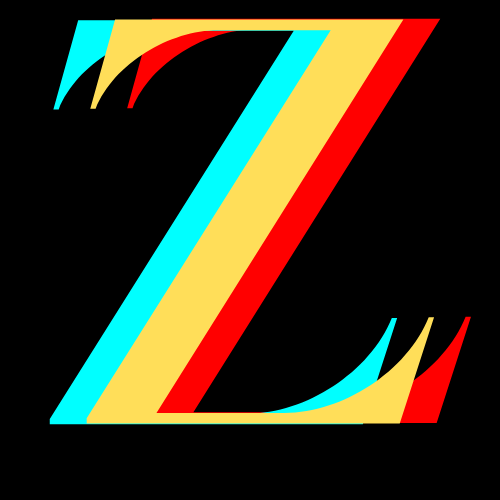